Affiliate Disclaimer
As an Amazon Associate I earn from qualifying purchases. It helps me keep the website going. Thank you for your support.
Equus ferus caballus, AKA the domestic horse, comes in many coat colors and coat patterns, but have you ever wondered just how these colors, and coat patterns, happen? If so, welcome to the fascinating world of horse color genetics!
Like most things, or actually, everything when it comes to living organisms, horse color comes down to pure genetics and genetic inheritance.
Granted, sometimes there seems to be an element of unpredictability about it – like when 2 bay parents produce a chestnut foal – but there is always a genetic explanation. The DNA that allowed that chestnut foal to happen is always lurking within the DNA of the parents.
In fact, color genetics is rarely if ever an uncontrolled lottery. Sure, there may be any number of possibilities but 99.99% of the time those possibilities are controlled by whatever available DNA there is in the parental mix. As for that 0.01%…well, that’s how new genetic variants happen!
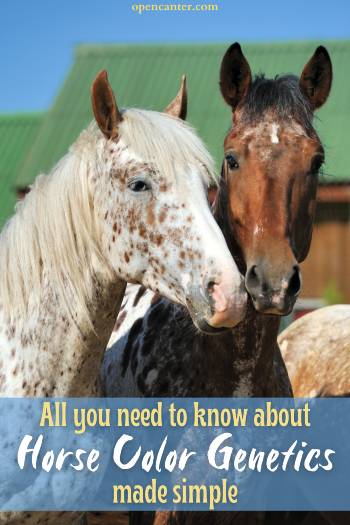
How Much Do We Understand About Horse Color Genetics?
We’ve come a fair way in our understanding of horse color genetics, particularly over the past few decades.
However, science and color genetics experts are still a LONG way from knowing all there is to know about equine coat colors! In fact, it’s probably fair to say we’ve only touched the tip of the iceberg.
We still don’t know, for example, things like how ‘flaxen’ and ‘sooty’ happen, and what exactly causes all the different shades of bay and chestnut. We have some ideas but we’re a long way from knowing for sure.
Indeed, the more we learn about horse color genetics, the more we realize we don’t yet know. Nevertheless, here are some of the things we do know about genetics in general, and about horse colors…
Horse Color Genetics 101 – Basic Terminology And Concepts
Genetics can be very confusing, even for those of us who understand the basics. So… before we go any further, we’ll try to make it slightly less confusing by explaining some basic genetic and horse color genetics terminology and concepts!
DNA:
Most DNA is found in the nucleus of cells and consists of 2 long strands of nucleotides that pair up like ladder rungs and twist together to form a spiral ladder called a helix.
Chromosome:
A long strand of DNA located within the nucleus of a cell. Chromosomes contain a series of genes along its length. Equus caballus (the domestic horse) has 32 chromosome pairs for a total of 64 chromosomes.
Nucleotide:
DNA is made up of four repeated building blocks called nucleotides which consist of a base (one of four chemicals: adenine (A), thymine (T), guanine (G), and cytosine (C)) plus a molecule of sugar and a molecule of phosphoric acid. The sugar and phosphoric acid molecules form the spine, or legs of the ladder whilst the 4 chemicals individually form half a ‘ladder rung’.
Base Pairs:
The nucleotides attach to each other (A always pairs with T, and G always pairs with C) to form chemical bonds called base pairs. This bonding completes the ‘ladder rungs’ connecting the two nucleotide strands.
Codon / amino acid:
A codon is a sequence of three base pairs, which codes for an amino acid, a unit of genetic code in a DNA strand. Amino acids are the ‘words’ used to compose DNA sentences if you like. For example, in a sentence like ‘the cat ate the rat’, each letter is a base pair, each word is a codon or amino acid, and the sentence created by the series of words is a DNA strand. There are 20 amino acids in total.
Gene:
A specific location on a chromosome where the DNA coding for a particular characteristic is located. Genes are the basic physical unit of inheritance because they hold the blueprints for producing all the various proteins that go into making things ‘work’.
Allele:
The actual DNA coding (blueprint) located at a gene. This code determines the appearance of the characteristic controlled by that gene; it may be either the wild-type coding or altered coding (a variant) that has been created through changes to the sequence of DNA coding. These altered sequences of DNA coding at a gene are responsible for the wide range of characteristics we see within species.
Wild type and wild type allele:
The original unaltered version of DNA coding of a gene
Genetic mutation:
An alteration of DNA sequencing within a gene. Has been replaced by the term ‘genetic variant’ unless the alteration causes a disease
Genetic variant:
The current terminology for non-disease causing genetic mutations. Taking our 3-letter word sentence above, we can see what happens when there is a typo that changes the sentence to ‘the sat ate the rat’. Now it doesn’t make sense, which is similar to what happens with genetic variants and mutations. A variant of a gene that doesn’t make ‘genetic’ sense results in a non-functioning protein. This can change the characteristic controlled by the ‘sentence’.
Genotype:
What an organism is genetically (internally or what you can’t see)
Phenotype:
What an organism is visibly (externally or what you can see)
Melanoblasts:
Melanocyte precursor cells
Melanocyte:
Pigment-producing cell
Melanosome:
Vessel or container within the melanocyte in which pigment is synthesized and stored
Melanin:
Pigment
Melanogenesis:
The process by which pigment is produced
Tyrosinase:
A copper-dependent enzyme produced by the TYR gene in melanocytes that triggers pigment production
Gamete:
A reproductive gene that contains only 1 copy of each gene in the genome. At conception, the gametes from each parent pair up to create a new set of gene pairs (and their associated alleles) in the offspring.
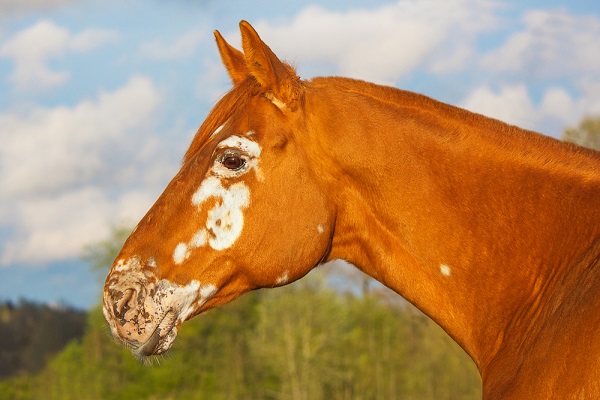
Understanding Genetic Inheritance
All genes, and thus alleles (except gametes) come in pairs, one inherited from each parent.
Traits created by variations in the DNA coding (alleles) at the genes responsible for those traits are also passed down from parent to offspring.
Alleles passed down from parents may result in a different phenotype in the offspring to that of the parents.
However, the alleles to produce those phenotypes are always carried by the parent/s. This means that 2 bay parents with the genetic makeup of Ee Aa can produce an ee aa (chestnut) or EE aa (black) offspring as well as bay offspring. That brings us nicely to…
Dominant And Recessive Alleles
Alleles are either dominant or recessive.
A dominant allele of a gene generally masks or hides a recessive version or allele of that same gene. So, if a copy of both a dominant and a recessive allele are present in a gene, the dominant allele will be the one expressed, or visible in the phenotype.
For a recessive allele to be expressed (visible in the phenotype), both copies of the alleles at that gene must be recessive i.e. the individual must be homozygous for that recessive allele.
Understanding Homozygosity And Heterozygosity
When an individual carries two identical alleles of a gene, they are ‘homozygous’ at that gene. It may be a pair of dominant alleles or a pair of recessive alleles.
When an individual carries two different alleles of a gene, they are ‘heterozygous’ at that gene.
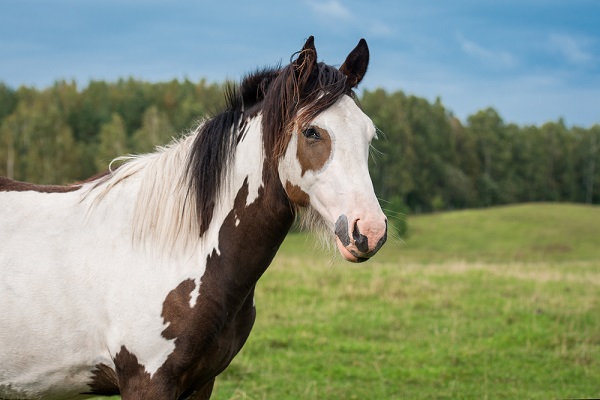
The World Of Melanocytes And Melanosomes
Before we (finally) move on to the basic principles of horse color genetics, and how horse color ‘works’, we will do a quick rundown on the ‘equipment’ that produces coat and skin color in mammals like horses, namely melanocytes.
Melanocytes are purpose-built melanin or pigment-producing cells. Their job is to synthesize raw materials, like the copper-dependent enzyme tyrosinase, into pigment. This process is controlled by a number of genes that control things like:
· What pigment color melanocytes produce,
· Transporting raw materials to the melanocytes,
· Moving raw materials across the melanocyte cell membrane,
· Ferrying the pigment through the various manufacturing stages within the melanocyte,
· Controlling the internal pH of the melanocyte and so on.
Variants in these genes can alter the way these processes work. In turn, these modify the pigment that is produced, and that brings us to…
Creating Different Horse Coat Colors
When the normal processes required to produce pigment are disrupted or changed, it results in diluted pigments. This means black pigment is produced where there should be red pigment, or the inability to produce black pigment at all. Welcome (once again) to the colorful, complex world of horse color genetics!
For example, palomino, buckskin, and the double cream dilute colors are produced by a variant of the MATP or Membrane Associated Transporter Protein gene. This gene produces a protein whose responsibility is delivering tyrosinase to stage II of the melanin biosynthesis process.
The variant of the gene that creates the cream dilutes produces a faulty or ‘rogue’ protein that transports tyrosinase and other compounds OUT of the cell instead of delivering them correctly. This reduces pigment production and creates the typical diluted pigment phenotype of cream dilutes.
Note: although smoky blacks also carry this variant of the MATP gene, a single copy of the variant can only modify red pigment. Why this is the case is one of those ‘mysteries’ we’re yet to uncover!
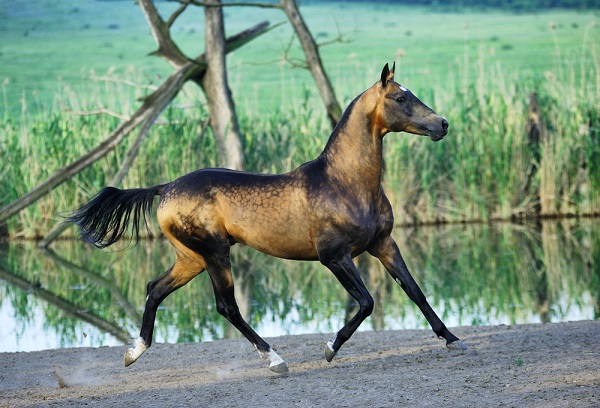
Horse Coat Patterns And How Melanocytes Get To Where They Need To Be
Coat colors are only one aspect of horse color genetics though. The other aspect is the various coat patterns. Although they also involve melanocytes and gene variants, the processes by which they occur (and the genes involved) are different from those that create coat colors.
This is why horses can have a modified coat color and a coat pattern, like a palomino tobiano.
Melanocytes start life as melanoblasts, which originate in neural crests along what eventually become the spine of the developing fetus. The melanoblasts migrate to various parts of the fetus, where they develop into melanocytes.
This migration and distribution process happens during early embryonic development. This is influenced, or controlled, by several genes, including the KIT and MITF genes.
If there are genetic variants present in these genes, it disrupts this migratory process. This leaves parts of the body (external and internal) without melanocytes.
Externally, this results in areas of unpigmented skin and hair (pink skin with overlying white hair) AKA coat patterns and white markings.
Internally, variants of some genes can have disastrous results, like lethal white overo, or can cause ocular and auditory problems (night blindness, deafness, etc) but that’s a story for another day!
The Basic Principles of Horse Color Genetics
And now, finally, with all that out of the way, the basic principles that underpin horse color genetics!
1. The ancestral color of all domestic horses is bay dun, the characteristics of which are controlled by the TBX3 gene. Our glossy evenly pigmented horses didn’t happen until around domestication and are caused by a variant of this gene we call ‘non-dun 2’ or nd2.
It’s also highly likely that most horse colors and coat patterns, with the exception of Lp (leopard spotting), likewise occurred after domestication and probably on non-duns. Ancient cave paintings depicting spotted horses alongside duns indicate Lp likely arose before domestication. Primitive spotted horses were also almost certainly duns.
2. There are only 2 pigment colors in horses:
· Black (eumelanin)
· Red (pheomelanin)
3. Equine melanocytes produce red pigment by default and have to be ‘switched over’ to produce black pigment.
4. There are only 3 base colors in horses:
· Bay (brown is a phenotype of bay and is more correctly called ‘dark bay’)
· Black
· Chestnut (red)
5. All horses are one of these 3 base colors.
6. The 3 base colors are produced by various combinations of alleles at 2 genes:
· The MC1R (melanocortin 1 receptor or Extension) gene (E or e) which produces a protein designed to switch on black pigment production in melanocytes, and
· The ASIP (agouti signaling protein or Agouti) gene (A or a) which produces a protein that decides which melanocytes can be switched on to produce black pigment
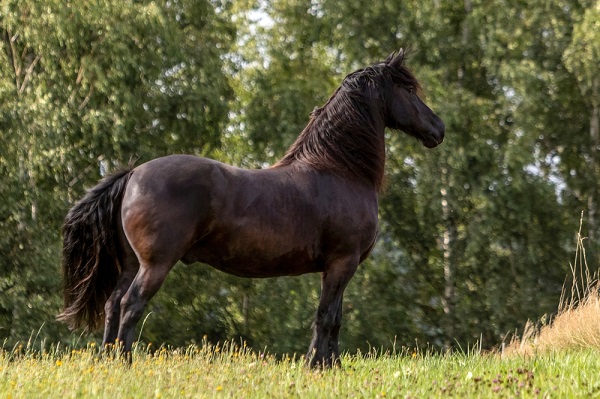
The Order Of Equine Base Color Dominance:
· Bay (E_A_) is dominant to both black and chestnut because of the presence of at least one copy each of dominant extension (E) and dominant agouti (A). Remember a dominant allele always masks a recessive allele so will be the one visible on the outside of the organism.
· Black (E_aa) is recessive to bay because of the presence of 2 copies of recessive agouti ‘aa’ but dominant to chestnut because of the presence of at least one copy of dominant extension ‘E’.
· Chestnut (ee _ _) is recessive to both bay and black because of the presence of 2 copies of the recessive extension ‘ee’. Because chestnuts can’t produce black pigment, agouti is inactive.
How Color Inheritance Works
Chestnut to chestnut always produces chestnut. This is because both carry 2 copies of the recessive extension allele so can only pass on a copy of this recessive allele to their offspring.
Any horse with a chestnut parent automatically has a copy of recessive extension ‘e’ because said chestnut parent only has a recessive extension to pass on.
Black to black can only produce black or chestnut, depending on which alleles of Extension are present because they only have recessive agouti (a) to pass on and bay requires at least one copy of dominant agouti (A).
Any horse with a black parent automatically has a copy of recessive agouti ‘a’. This is because a black parent only has recessive agouti to pass on.
The Role Of Genes In Determining Equine Coat Color
The 3 base colors can be modified by variants of genes involved in pigment production. As mentioned earlier, to produce the various dilute colors. These genes include the:
· MATP gene (cream, pearl, and sunshine),
· PMEL17 gene (silver),
· SLC36A1 (champagnes),
· MFSD12 gene (mushroom),
Note: although the TBX3 gene also modifies pigment production, the original version or wild type of this gene produces dun (see above), whilst the variants nd1 (non-dun 1) and nd2 (non-dun 2) produce what we now consider ‘regular’ coated horses i.e. non-duns.
Other genes modify melanocyte distribution to create coat patterns and white markings.
· KIT gene (roan, sabino 1, white spotting, and tobiano),
· MITF gene (splashed white 1, 3, 5, and 6),
· PAX3 gene (splashed white 2 and 4),
· ENDRB gene (frame overo or overo lethal white),
· TRPM1 gene (Lp or leopard complex),
· RFWD3 gene (PATN)
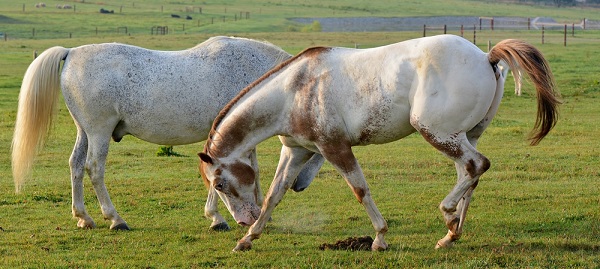
Dispelling A Few Common Misconceptions And Incorrect Horse Color Genetics Terminology
Unfortunately, even with the increasing amount of information now available, common misconceptions and ‘sayings’ about equine color genetics persist.
One of these is the use of terms like ‘bay gene’, ‘black gene’, or ‘red gene’. There’s no such thing… Rather, and to re-iterate (because this is fundamental to understanding horse color genetics):
The various alleles of the MC1R and ASIP genes work together to create the 3 equine base colors. There are no ‘bay’, ‘black’, or ‘red’ genes per se.
All other equine coat colors, and all coat patterns, are caused by variant alleles of genes that either:
· Play a role in the manufacture of melanin, or
· Are involved in the migration and distribution of melanocytes during fetal development.
Throwbacks Are Not A ‘Thing’ In Genetics
Genes and alleles do NOT SKIP generations and there are no such things as “THROWBACKS” in genetics.
De Nova Or Spontaneous DNA Variants Do Happen
The ONLY time you will find DNA, or alleles, in an offspring that is not present in either parent is with a DE NOVA or SPONTANEOUS variant.
These are a ‘typo’ that happens in the DNA coding at a gene during conception or shortly afterward, which creates a new allele of that gene in the individual in which the variant occurred.
That individual can then pass on that new allele to its offspring, which is how genetic variants become entrenched in a species. Some genes, like the KIT gene, are very prone to genetic ‘typos’.
All Horses Carry ALL Equine Genome Genes
Although the phrases ‘doesn’t carry agouti’ or ‘doesn’t carry extension’ are generally meant to indicate a horse has 2 recessive agouti/extension gene alleles respectively, it isn’t correct terminology.
Agouti and extension both refer to genes (ASIP and MC1R respectively) and all organisms, including horses, have a complete genome for their species. That means they have a full complement of genes (and gene pairs) including agouti and extension genes.
However, just to confuse the issue, it is fine to say a horse does or doesn’t ‘carry cream’ (or any one of the other dilution and coat pattern alleles). This is because these names don’t refer to genes but to DNA code.
A horse may or may not have this particular version of an allele at a gene. But – if you were to say the horse ‘doesn’t carry MATP’ (the gene responsible for cream et al), that’s the same as saying ‘doesn’t carry agouti/extension’ because you’re now referring to the gene, not the DNA code.
Correct Horse Color Genetics Terminology
· A horse has dominant and/or recessive alleles at their extension and agouti genes
· EE is correctly referred to as being ‘homozygous for dominant extension alleles’ NOT ‘homozygous for black’.
· ee is correctly referred to as being ‘homozygous for recessive extension alleles’ NOT ‘homozygous for red’, ‘homozygous for chestnut’, ‘doesn’t have extension’, or any of the other terms that get used.
· AA is correctly referred to as being ‘homozygous for dominant agouti alleles’ NOT ‘homozygous for bay’.
· aa is correctly referred to as being ‘homozygous for recessive agouti alleles’ NOT ‘homozygous for black or non-bay’, ‘doesn’t have agouti’, ‘doesn’t have bay’ etc.
Final Thoughts About Horse Color Genetics
Hopefully, you’re now a little bit wiser about horse color genetics! If not, don’t worry. You don’t ride the color, and a good horse is never a bad color. Trite but true! Color genetics is important to some horse owners, and not so important to others. Furthermore, your horse certainly doesn’t care what color it is, or how it came by its color.