Affiliate Disclaimer
As an Amazon Associate I earn from qualifying purchases. It helps me keep the website going. Thank you for your support.
To the average layperson, the importance of horse color genetics is often a baffling mystery. Does it really matter if a horse is bay, black, or chestnut? You don’t, after all, ride the color! Or to paraphrase yet another saying – a good horse is never a bad color. So why is there such an intense focus on coat color-related disorders in horses, like Overo Lethal White Syndrome?
Understanding coat color-related disorders in horses is an increasingly ethical consideration for breeders of colored equines. Some of these disorders come with serious and even fatal health consequences for the horse. Overo Lethal White Syndrome and Coat Color Dilution Lethal are cases in point, as the term ‘lethal’ probably indicates.
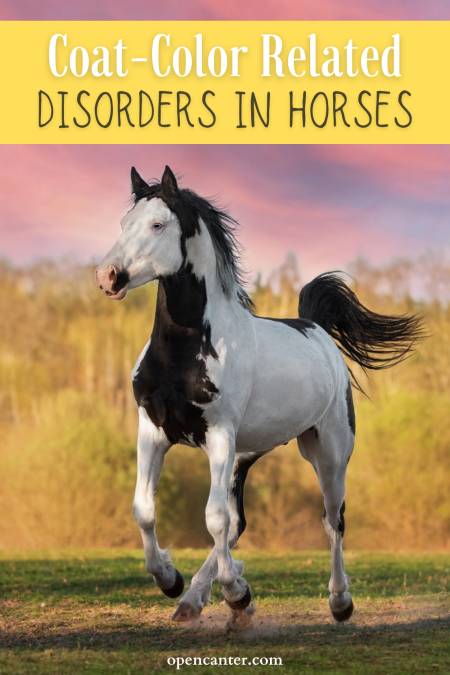
Overo Lethal White Syndrome and Understanding Horse Colour Genetics Important?
We all love pretty-coloured horses – patchy, spotty, iridescent gold, dapple-grey… So, why are some of these lovely colors and coat patterns associated with health disorders?
The reason for this, and indeed for many coat color-related disorders in horses, humans, dogs, cats, mice, and other species is that pigment is important for more than just coat and skin color. It performs a range of functions in other organs as well.
The Interrelationship Between Pigment And Organ Function
The role of melanocytes in the skin and coat is well known because they produce the various colors and coat patterns seen on our horses and ponies. Their role in other organs however is not so obvious, or well known.
· Pigment in the inner ear helps absorb excess sound and protects its delicate structures from sound-related damage.
· Pigment gives the retina its color plus provides a range of protective functions. The RPE or retinal pigment epithelium is a thin layer of tightly packed cells full of melanin. It absorbs excess light, recycles photoreceptor cells, and transfers nutrients from underlying blood vessels to the rods and cones (photoreceptor cells) in the retina.
Disorders that affect pigment in other species (i.e. albinism) produce a range of ocular conditions that are directly related to pigment reduction in these structures. Note – true albinism is not found in horses.
· Melanin in the brain is believed to perform a neuroprotective role. Notably, Parkinson’s disease is associated with a loss of melanin-containing neurons in the substantia nigra. [source]
Embryonic Cellular Processes Are Tightly Regulated
Melanoblasts (the precursors of melanocytes, or pigment-producing cells) and other precursor cells form in neural crests – groups of temporary embryonic cells – along the neural tube (which eventually becomes the spine of the developing embryo). From there, they migrate throughout the fetus to where they need to go.
These migratory and development processes are tightly regulated and controlled by a series of genes. This is meant to ensure the immature cells get to where they need to go, in good working order, and develop into fully functional mature cells. Some of the genes involved in melanocyte processes include:
· KIT (Receptor Tyrosine Kinases) gene
· MITF (Microphthalmia-Associated Transcription Factor) gene
· PAX3 (Paired Box Gene 3) gene
· EBDRB (Endothelin Receptor Type B) gene
· TRPM1 (Transient Receptor Potential Cation Channel Subfamily M Member 1) gene
· RFWD3 (Ring Finger and WD Repeat Domain 3) gene
· MYO5A (Myosin VA) gene
· STX17 (Syntaxin-17) gene
· PMEL17 (Premelanosomal Protein 17) gene
Some of these genes are only involved in melanocyte function. Others are involved in various other regulatory and signaling processes as well but more on that later.
The Effect Of Mutations In Genes Involved in Melanocyte Processes
Normal pigment migration and function require ‘normal’ copies of genes like those mentioned above. When these genes have variations from the norm (either mutations or variants), it can disrupt the processes they control or are involved in.
Some disruptions are obvious because they affect phenotype. Disruptions in skin melanoblast migration for example can leave parts of the skin without melanocytes, resulting in unpigmented areas with corresponding unpigmented coat hair (pink skin and white hair).
Other disruptions are not so obvious because they affect melanocyte migration or pigment development in internal organs, and can affect the function of those organs in significant ways, sometimes lethally.
Variants and mutations in the following genes produce coat patterns, some of which are associated with coat color-related disorders in horses:
· KIT gene (sabino, white spotting, roan, tobiano)
· MITF gene (splashed white 1, 3, 5, and 6)
· PAX3 gene (splashed white 2 and 4)
· EBDRB gene (Frame overo)
· TRPM1 gene (Lp or leopard complex)
· RFWD3 gene (PATN)
Mutations in the genes below alter pigment-processing pathways and are all associated with coat color-related disorders in horses:
· PMEL17 gene (Silver)
· MYO5A gene (Lavender)
· STX17 gene (grey)
Mutation Vs. Variant
You’ve probably noticed the use of the terms ‘mutation’ and ‘variant’ above. These terms are often used interchangeably but they mean different things:
· A mutation is a version of a gene that results in a disease, or disorder. Examples are Overo Lethal White Syndrome, Lavender Foal Syndrome, grey, Congenital Stationary Night Blindness, etc.
· A variant is a version of a gene that either has no significant effect or doesn’t have an adverse effect, on the health of the organism. Examples are the variants of the ASIP (agouti) and MC1R (extension) genes that produce the 3 equine base colors, and variants in genes that result in dilutions like cream and champagne.
Pleiotropy And The Pleiotropic Effect
Some genes are involved in multiple regulatory processes. Others are involved in one regulatory process that affects several organs. Either way, they can influence multiple traits, characteristics, or organs in sometimes unexpected or apparently unrelated ways.
This is the pleiotropic effect – where one gene affects the expression of two or more other genes, and/or can alter the function or appearance of several organs.
Some better-known examples of pleiotropy involve instances where the alteration of color and skin pigment is secondary to the main function of the gene involved. In other cases, the alteration of pigment processing pathways causes issues in other organs that also rely on pigment to function correctly.
Pleiotropy At Work In Coat Color-Related Disorders In Horses
Some of the equine gene mutations and variants that create popular coat colors and coat patterns also result in pleiotropic diseases. Known examples of coat color-related disorders in horses include:
1. A mutation in the TRPM1 gene that causes the Leopard Spotting pattern also causes Congenital Stationary Night Blindness (CSNB) in horses that carry 2 copies of the mutation,
2. Some of the MITF and PAX3 gene variants are mutations in that they can also cause deafness along with the striking splashed white coat patterns,
3. Some of the KIT gene variants are thought to be embryonic lethal when inherited in double copy because there are no known living homozygotes for these variants,
4. A double copy of the mutation in the PMEL17 gene that creates the silver coat dilution also causes multiple congenital ocular anomalies (MCOA),
5. Grey is caused by a doubling up of DNA code close to the STX17 gene that results in increased pigment production and ultimately the development of internal and external melanomas,
6. A double copy of a mutant protein produced by the MYO5A gene produces Coat Colour Dilution Lethal (CCDL), a light or ‘lavender’ colored foal with severe neurological symptoms,
7. Finally, there is frame or Overo Lethal White Syndrome, a mutation of the ENDRB gene that, when inherited in double copy, produces a lethal white foal that has to be euthanized shortly after birth.
1. Lp, The TRPM1 Gene, And Congenital Stationery Night Blindness
Lp, or Leopard Complex, is a variant of the TRPM1 gene that acts as a switch to make the white blankets (PATN) associated with the appaloosa coat pattern visible. It also causes the characteristics associated with the color – mottled skin, striped feet, and progressive depigmentation (varnishing) across the soft tissue areas of the body.
The TRPM1 Gene And Coat-Color-Related Disorders In Horses
The TRPM1 gene is a member of the transient receptor potential (TRP) channel family. These genes produce channeling proteins that let positively charged ions (like Ca2+) flow into hyperpolarised cells to depolarise them. Hyperpolarised cells must be depolarised to transmit signals properly.
Notably, TRMP1 is involved in signaling pathways that affect early melanocyte migration and survival. High levels of TRMP1 proteins are associated with more pigment and darker skin whilst lower levels of TRMP1 proteins are associated with lighter skin and less pigment. [source]
Similarly, the skin cells of depigmented LP positive horses contain significantly fewer TRPM1 proteins than those of non-LP horses [source], resulting in the gradual coat and skin depigmentation that characterizes LP horses. Homozygotes have fewer TRPM1 proteins again and typically end up totally depigmented.
The TRPM1 Gene And Congenital Stationery Night Blindness
The TRPM1’s primary function, however, is not about melanocytes so much as it is about helping us see in the dark! Retinal tissue is in fact far richer in TRPM1 proteins than skin cells.
How We See In The Dark
Eyes have 2 types of retinal photoreceptor cells – cones and rods. In broad daylight or when there is plenty of light, the cone photoreceptors control vision. These cells communicate with the brain via various pathways to produce colored and daytime vision. At night, or when light conditions dim, rod photoreceptor cells take over and the ‘night vision’ system kicks in.
Night vision involves 2 types of light-sensitive cells – rod photoreceptors (rod cells) and a secondary type of neural cell called rod ON-bipolar cells (bipolar cells). These cells work together to allow us, and horses, to see in dim light. Here is a ‘simple’ rundown of the process to explain how LP is a coat-color related disorder in horses:
1. When light fades, the rod cells activate and…
· Start producing glutamate, a neuro-transmitting chemical released in response to reducing levels of light. This is a directly inverse process – the less light there is, the more glutamate the rods produce. When there is no light at all, glutamate production reaches full capacity.
· Begin to convert the light into electric signals that are passed on to the bipolar cells.
2. Depolarised bipolar cells forward these signals to ganglion cells, which send them on to the brain for translation into what we’re seeing.
3. Bipolar cells switch between hyperpolarised and depolarised states BUT only work when depolarised. This switching process is controlled by the glutamate. However, the more glutamate there is, the more hyperpolarised the bipolar cells become until they stop working. When that happens, we stop seeing in the dark, or at least we would without the TRPM1 gene and its protein!
In retinal tissue, TRPM1 genes are located in the bipolar cells and their channeling protein allows positively charged ions to enter hyperpolarised bipolar cells and depolarise them. They are triggered when glutamate molecules bind to special receptors on the surface of the bipolar cells.
Horses with 2 copies of the TRPM1 variant that causes the Lp spotted coat pattern can’t produce a correctly functioning TRPM1 protein so are unable to depolarise their retinal bipolar cells. The cells thus remain hyperpolarised, unable to send off signals to be decoded into vision by the brain and result in a horse (or human) that can’t see in the dark. This is Congenital Stationery Night Blindness (CSNB).
The same mutation is also responsible for the reduced level of TRPM1 proteins in the skin and coat that leads to the depigmentation of skin and coat in LP carriers.
2. Splashed White, The MITF And PAX3 Genes, And Deafness
Structures within the inner ear require melanin to develop and function correctly. Reduced melanin in these structures is associated with hearing loss (melanin protects against damaging excess sound energy), whilst an absence of melanin (due to a lack of melanocytes) is associated with congenital deafness.
The MITF And PAX3 Genes And Coat-Color Related Disorders In Horses
The various splashed white phenotypes are caused by mutations in the MITF (SW1, 3, 5, 6) and PAX3 (SW2, 4) genes. Splashed white horses typically have pigment across the top half of the body with a white bottom half, as though the horse has waded through white paint. However, as with most of these variants, expression varies.
· The MITF gene produces proteins that are required for normal melanocyte function. Notably, it activates various other genes involved in regulating melanocyte migration and propagation.
· The PAX3 gene has a dual role as it’s involved in melanoblast migration and distribution, as well as the development of certain types of nerve tissue. In addition, it interacts with/can affect the function of the MITF gene.
These 2 genes are responsible for a host of regulatory functions with respect to melanoblast/melanocyte development and function.
The MITF And PAX3 Genes And Deafness
With respect to these genes and coat-color related disorders in horses:
· Researchers have found strong correlations between MITF gene variants and reduced pigment/lack of pigment in inner ear structures with corresponding loss of auditory function. [source]
· Horses with the MITF variant that causes SW3 can be deaf if the absence of pigment is located ‘inside their ears’.
· Individuals with the PAX3 variant SW2 can also be deaf, perhaps because the interaction between PAX3 and MITF potentially affects the function of MITF and thus impedes the development of inner ear melanocytes needed for auditory function.
3. White Spotting, The KIT Gene, And In Utero Lethal Pregnancies
Two copies of some of the white spotting variants of the KIT gene have not been found in living horses, leading researchers to suspect that these particular variants may be in utero lethal in homozygotes.
Melanin plays an integral role in many internal organs, including the brain, liver, lungs, etc. It also supports and stiffens heart muscles.
Thus the absence of, or even severely reduced levels of melanin in these structures caused by 2 copies of depigmenting KIT gene variants may result in non-viable embryos that are reabsorbed or aborted early in pregnancy.
4. Silver, The PMEL17 Gene, And Multiple Congenital Ocular Anomalies
Mutations in genes responsible for pigment production can cause ocular coat-color-related disorders in horses. This is because structures within the eye contain melanocytes that are affected by these mutations.
The PMEL17 Gene And Coat-Color-Related Disorders In Horses
The silver coat color in horses is caused by a mutation in the melanosome-specific PMEL17 gene. This gene provides the instructions for producing a pivotal melanosomal protein that plays a key role in the structural organization of immature eumelanin (black pigment) producing melanosomes.
PMEL17 is a remarkable protein that starts life as a transmembrane protein before transforming into internal fibrillar sheet structures in melanosomes transitioning from stage 1 to stage 2. These fibrillar structures form the framework, or scaffolding, upon which black pigment is deposited during stages 3 and 4 of melanosome development.
It is believed the silver mutation in PMEL17 affects how black pigment is laid down on these fibrillar sheet structures, resulting in changes to the appearance of black pigment in individuals carrying the variant i.e. silver dilutes.
As PMEL17 proteins are also involved in pigment development in ocular structures, the silver mutation causes a range of eye irregularities referred to as multiple congenital ocular anomalies (MCOA). These include ocular cysts, enlarged cornea, abnormal retinal development, and various other ocular abnormalities.
Although this condition in heterozygotes is typically non-discernible and doesn’t cause any issues with their health or vision, homozygotes can display a range of symptoms such as:
· Retinal detachment,
· Retinal dysplasia,
· Cataracts,
· Protrusive corneas (pop eyes),
· Dysfunctional pupils that don’t have normal responses,
· Myopia
However, not all homozygotes exhibit these symptoms. It’s also not clear exactly how the disruptions in pigment development contribute to these symptoms.
5. Grey, The STX17 Gene, And Melanomas
From the famous Windsor Greys to the white Lipizzaner stallions, and wild Camargue horses – grey horses have been singled out for their color ever since the first one was born way back when. The color though, which isn’t actually a color but a gradual depigmentation of coat hair, is caused by a mutation close to the STX17 gene.
The STX17 Gene And Coat-Color Related Disorders In Horses
Greying in horses comes about because of a duplication of DNA code in a non-coding section of DNA close to the STX17 gene. This section of DNA contains a binding site for MITF proteins.
If you recall, the MITF gene is heavily involved in melanocyte migration and distribution. Although the duplication of DNA doesn’t affect the protein produced by the STX17 gene, the duplication of the MITF binding site does.
Horses that carry 1 copy of the mutation have an extra copy of the MITF binding site whilst those with 2 copies have an additional 3 MITF binding sites at that location.
That means they bind twice / 4 times as much MITF protein respectively. This exponentially intensifies melanocyte migration and growth, resulting in the typical hyper-pigmented phenotype of a grey foal.
Although the exact mechanism that causes the depigmentation is not currently confirmed, it’s thought that the up regulating of pigment production causes a premature ‘burn out’ of melanocyte stem cells required for ongoing coat pigmentation.
Unfortunately, the intensified melanocyte growth also causes melanomas, and not just in the skin. Indeed, these melanomas can occur wherever there are melanocytes, including within internal organs.
Homozygous greys also go grey faster and have a far greater chance of developing melanomas than heterozygous greys.
6. Lavender, The MYO5A Gene, And Coat Colour Dilution Lethal (CCDL)
A double copy of a mutant protein produced by the MYO5A gene produces Coat Colour Dilution Lethal (CCDL), a light or ‘lavender’ colored foal with severe neurological symptoms: muscle tremors, hyperextension of neck and head leading to an inability to suckle, uncontrolled eye movements, and paddling legs that prevent them from standing properly.
Also called Lavender Foal Syndrome (LFS), it’s found in horses with Arabian breeding. Humans have a similar disorder called Griscelli syndrome.
Heterozygotes with only 1 copy of the mutation have normal pigment and neurological function.
The MYO5A Gene And Coat-Colour Related Disorders In Horses
The MYO5A gene produces myosin V, a protein involved in intracellular trafficking i.e. the transport of various cellular components within cells.
In particular, it transports components required for correct neurological communication and function. However, it is also probably involved in transporting mature melanosomes to the melanocyte cell membrane for transfer to the keratinocyte, hence its effect on coat and skin pigment. [source]
Mutations in the gene, like the one that causes CCDL in horses, result in dysfunctional proteins that can’t transport their ‘cargo’ correctly. This leads to the types of neurological symptoms and diluted pigment seen in ‘Lavender Syndrome’ foals. These foals, like foals with the Overo Lethal White Syndrome, are usually euthanized.
7. Frame, The ENDRB Gene, And Overo Lethal White Syndrome
Frame overo is a very popular coat pattern characterized by horizontal white markings along the center of the torso and up the neck ‘framed’ by the base color. It is caused by a mutation in the EDNRB gene.
The ENDRB Gene And Coat-Colour Related Disorders In Horses
This gene belongs to a group of genes involved in regulating cell propagation and differentiation. Notably, it codes a protein involved in the developmental regulation of neural crest cells destined to become enteric ganglia cells (intestinal nerves) AND melanocytes. Intestinal ganglion cells control the muscular contractions that push contents through the intestines.
The mutation that causes Overo Lethal White Syndrome results in a defective protein that disrupts this process. The result in homozygotes of the mutation is a pure or nearly pure white coat and an absence of ganglion (nerve) cell development in the large intestine (intestinal aganglionosis). A similar mutation in humans causes Hirschsprung’s Disease. Lethal white foals have to be euthanized.
Heterozygotes still have normal intestinal ganglion cell development but may exhibit some depigmentation, resulting in the frame overo phenotype.
Overo Lethal White Syndrome and Other Coat-Colour Related Disorders In Horses
Knowing the mutations and changes in cellular DNA that cause the various coat patterns and colors, and their associated diseases, like Overo Lethal White Syndrome, helps horse breeders make informed and ethical breeding choices.
Then there is the simple fact that in some markets, producing a particular color guarantees a sale whilst not producing it may result in a culled foal! Once again, knowing how to produce the desired color reduces the risk of producing that ‘unwanted color’ foal.